the Creative Commons Attribution 4.0 License.
the Creative Commons Attribution 4.0 License.
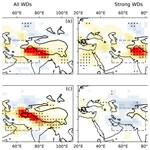
Increasing frequency and lengthening season of western disturbances are linked to increasing strength and delayed northward migration of the subtropical jet
Kieran M. R. Hunt
Western disturbances (WDs) are cyclonic storms that travel along the subtropical jet, bringing the majority of seasonal and extreme precipitation to the Hindu Kush, Karakoram, and western Himalaya in the winter months. They are a vital component of the region's water security. Although typically most common in the winter, WDs can also interact with the summer monsoon, leading to catastrophic consequences. These seem to be happening more frequently, and along with increasingly harsh winter seasons, questions are now being asked about how climate change is affecting WD frequency and intensity in both summer and winter seasons.
An analysis of 17 previous studies assessing trends in WD frequency revealed no consensus, at least in part because they quantified trends in different regions, seasons, and time periods. In this study, a more robust approach is used, quantifying trends in WD frequency and intensity by region and month using a track catalogue derived from 70 years of European Centre for Medium-Range Weather Forecasts (ECMWF) reanalysis (ERA5) data. Winter WDs have increased significantly over the western and central Himalaya and the Hindu Kush in the last 70 years. This trend is attributed to a strengthening of the subtropical jet. The WD season has also significantly lengthened with WDs becoming far more common in May, June, and July, months where they were previously rare. For example, WDs have been twice as common in June in the last 20 years than during the previous 50. This is attributed to a delayed northward retreat of the subtropical jet, which historically has occurred before the onset of the summer monsoon. The most important implication is that the frequency of “monsoonal” WDs is increasing significantly, and therefore, due to climate change, catastrophic events like the 2013 Uttarakhand floods and the 2023 north India floods are becoming much more frequent.
- Article
(4497 KB) - Full-text XML
- BibTeX
- EndNote
Western disturbances (WDs) are upper-level troughs embedded in the subtropical westerly jet. Dynamical instabilities in the jet, often arising over the Mediterranean, grow baroclinically as they move eastwards, arriving as cyclonic winter storms over the Hindu Kush, Karakoram, and western Himalaya several days later (Singh, 1971; Dimri et al., 2015). On average, six to seven strong WDs pass over the Indian subcontinent each month during winter (Rao and Srinivasan, 1969; Hunt et al., 2018a).
Here, they are responsible for the majority of winter mean and extreme precipitation (Hunt et al., 2018b, 2019a). WDs affect Iran, Afghanistan, Pakistan, and India and occasionally extend their reach further east to Nepal and Bangladesh (Pisharoty and Desai, 1956; Rao and Srinivasan, 1969). However, their impact is most strongly felt over north India and the western Himalaya where they cause cold waves (Mooley, 1957; De et al., 2005), fog (Smith et al., 2023), avalanches (Ganju and Dimri, 2004), landslides (Hunt and Dimri, 2021), lightning (Unnikrishnan et al., 2021), and heavy precipitation through interaction with the orography (Singh and Agnihotri, 1977; Dimri and Chevuturi, 2014).
The importance of WDs lies in their contribution to winter precipitation, crucial for water security (Benn and Owen, 1998; Archer and Fowler, 2004; Thayyen and Gergan, 2010) and agriculture (Yadav et al., 2012). They therefore have direct and indirect economic impacts on India, Pakistan, and neighbouring countries (Dimri et al., 2015). Extreme rainfall events associated with WDs can also cause severe flooding, especially near the start and end of the Indian summer monsoon (Dimri and Niyogi, 2012). These interactions with the monsoon can be dynamical or thermodynamical in nature (Hunt et al., 2021), but the increased convective instability and moisture supply provided by the monsoon can result in catastrophic flooding, for example resulting in over 6000 deaths in Uttarakhand in June 2013 (Kotal et al., 2014; Chevuturi and Dimri, 2016) and over 100 in north India in July 2023 (Associated Press, 2023).
Several methodologies have been used to quantify WD variability or trends over the past century: direct counting from various weather reports, feature-based tracking in reanalysis data, utilisation of filtered variance or clustering of appropriate meteorological fields in reanalyses, or inference from winter weather records in the western Himalaya region.
Several studies, as depicted in blue in Fig. 1, have employed weather reports to compile WD counts (Das et al., 2002; Shekhar et al., 2010; Ahmad and Sadiq, 2012; Kumar et al., 2015; Midhuna et al., 2020; Ahmed et al., 2022). These tend to report a decline in WD frequency, although this is not universal, and several are not statistically significant. Other recent research (Cannon et al., 2016; Hunt et al., 2018a; Nischal et al., 2022; Javed et al., 2022) has used feature-tracking algorithms applied to reanalysis data to generate WD variability records for trend analysis, none of which showed statistically significant trends. These are depicted in red in Fig. 1. The third type of study, shown in green in Fig. 1, has typically used bandpass-filtered upper-level geopotential variance as a proxy for WD frequency (Cannon et al., 2015; Madhura et al., 2015; Krishnan et al., 2019; Neal et al., 2020). These findings have revealed mixed results, including both significantly positive and negative trends in WD frequency. The use of geopotential variance as a proxy should be approached with caution as it captures both WD frequency and intensity. Studies using this method tend to be more likely to report an increasing trend in WD intensity, unlike those focusing solely on WD frequency.
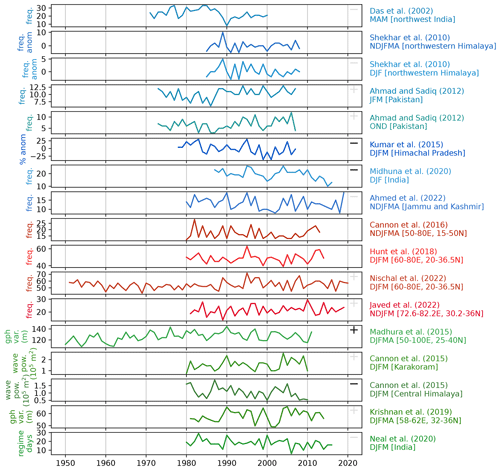
Figure 1Trends in WD activity since 1950 from the studies discussed in Sect. 1 that have available data. For each study, data are presented in the original units with the stated season and impact region given. Those in blue derive their time series from observational records, such as India Meteorological Department (IMD) bulletins; those in red use tracking techniques applied to reanalysis data; and those in green use variance-based methods applied to reanalysis data. For each time series, a black ± in the upper-right corner indicates that the trend is significantly different from zero at the 95 % confidence level; a grey ± is used for the 50 % confidence level.
There is considerable disagreement among these studies regarding the trend in WD frequency over the past 70 years, with the trend being sensitive to the region of study, the seasonal definition, the definition of WD used, and even the chosen dataset period. Other factors, such as trends in the subtropical jet, decreasing static stability, and substantial interannual variability in jet behaviour further complicate trend interpretation (Abish et al., 2015; Yuval and Kaspi, 2020; Hunt and Zaz, 2023).
The aim of this paper is to resolve these disagreements using a more robust analysis method relying on a database of WD tracks covering 1950 to the present day. We split the problem into four research questions:
-
Do trends in WD frequency vary spatially?
-
Do trends in WD frequency vary seasonally? If so, are WDs increasing in frequency in the pre-monsoon and monsoon seasons, extending their range beyond the winter months as hypothesised by Valdiya (2020)?
-
Can differences in trends be explained by different definitions of WDs, namely using different intensity thresholds?
-
What are the causes of robust trends in WD frequency?
The reanalysis and WD track data used are described in Sect. 2, the results are presented in Sect. 3, and the results are summarised and their implications discussed in Sect. 4.
2.1 ERA5
ERA5 is the fifth-generation atmospheric reanalysis of global climate produced by the Copernicus Climate Change Service (C3S) at the European Centre for Medium-Range Weather Forecasts (Hersbach et al., 2020). Data from ERA5 (available from https://cds.climate.copernicus.eu/cdsapp#!/home, last access: 28 February 2024) cover the entire globe on a 30 km grid and resolve the atmosphere on 137 levels from the ground up to 80 km in altitude. It covers January 1940 until the present day at hourly frequency. At reduced spatial and temporal resolutions, ERA5 also includes uncertainty information for all variables. We use both pressure-level variables from ERA5 for both WD tracking (see Sect. 2.2) and the computation of jet statistics.
2.2 Western disturbance track dataset
WDs are identified using the feature-tracking algorithm described in Hunt et al. (2018a). Relative vorticity is averaged across the 450–300 hPa layer and then spectrally truncated to T42 (∼300 km at the Equator) to remove high-frequency noise that hinders tracking. For each region of positive vorticity, the centroid is located and labelled as a WD candidate. These centroids are connected between time steps using a nearest-neighbour algorithm with a biased search to take into account the steering winds of the subtropical jet. Systems that do not on average travel eastward, last less than 48 h, or do not pass through the box (20–42.5° N, 60–80° E) are rejected.
Applied to ERA5, this gives over 70 years of track data (1950–2022). Although the ERA5 dataset starts in 1940, we only use data from 1950 onwards because upper-air soundings over central and south Asia were very sparse before this date (Lanzante et al., 2003), leading to large uncertainties in the reanalysis fields in our study region.
The method followed here is almost identical to Nischal et al. (2022) except the northern edge of the catching box is extended from 36.5 to 42.5° N to ensure that all WDs that potentially impact north India are included, and the spectral truncation is at T42 rather than T63 as this better captures broad-trough WDs with multiple vorticity maxima.
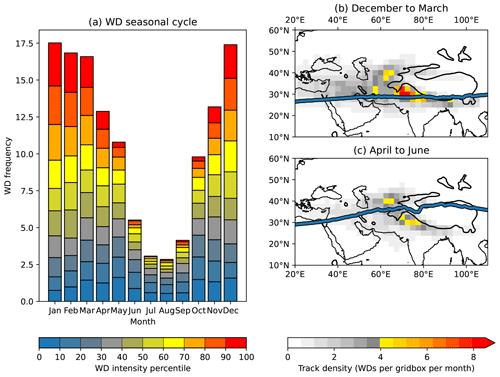
Figure 2The seasonal cycle of WDs, computed between 1950 and 2022. (a) Mean WD frequency for each month, computed as the number of WDs entering the region (20–40° N, 50–80° E,). These are further stratified by overall intensity percentile, where intensity is defined as the maximum value of 350 hPa ζ that a WD reaches during its lifetime. (b) Map of average WD frequency from December to March, computed as the number of unique WD tracks that enter a given 2.5°×2.5° grid box per month. The mean location of the subtropical jet is plotted in blue, computed for each longitude as the latitude during that season that has the highest mean 200 hPa zonal wind speed. (c) As (b) but for April to June. Smooth black lines indicate an orographic height of 2000 m.
We start by examining some mean statistics for WDs over the last 70 years (Fig. 2). The seasonal cycle of WD frequency (Fig. 2a) is extremely pronounced, with WDs being extremely common during the winter months (December to March), somewhat less common during the post-monsoon and pre-monsoon months (October to November and April to May respectively), and rather uncommon indeed during the summer monsoon months (June to September). WDs are about 8 times as frequent in January as in August. There is also a strong seasonality to their intensity. By stratifying the whole population into deciles of maximum vorticity (i.e. the peak intensity reached by a given WD during its lifetime), we see that the strongest WDs are proportionately far more common during the winter months. In contrast, during the monsoon most WDs are substantially weaker than the median. As a result, WDs reaching the top pentile of intensity are more than 50 times more common in January than in August.
Alongside variations in frequency and intensity, WDs also exhibit marked seasonality in their spatial distributions. Track density during the winter months (Fig. 2b) when the subtropical jet is at its lowest latitude is concentrated over the western Himalaya and southern Karakoram, with high frequencies also present over the central Himalaya and Hindu Kush mountains. As the jet recedes poleward, eventually passing north of the Karakoram during the pre-monsoon months, track density also migrates northwards, lessening considerably over north India but strengthening on the northern edge of the Tibetan Plateau and parts of the Karakoram. It also spreads out considerably, reflecting the greater variability in the subtropical jet behaviour before and after the winter season (April–May and October–November; Schiemann et al., 2009). Based on these maps, we assign a “WD box” (20–40° N, 50–80° E) that captures the highest density of WD tracks over south Asia. This will be useful for statistical purposes later as it also ensures that we only use tracks that are likely to have an impact on Pakistan, north India, or the mountain ranges around the western Himalaya.
Now that we are familiar with the mean and seasonal behaviour of WDs, let us investigate their trends. Since 1950 WD frequency over northern India has increased significantly (passing a 95 % confidence interval that the trend is nonzero), regardless of intensity (Fig. 3a and b). This includes the western Himalaya, the central Himalaya, and the Hindu Kush – winter WDs in these regions are becoming more frequent at a rate of about 20 % per century. There is no significant signal in winter WD frequency over the Karakoram, however, and this is perhaps surprising given that the theories explaining the Karakoram Anomaly1 require a substantial increase in snowfall over the region (de Kok et al., 2018).
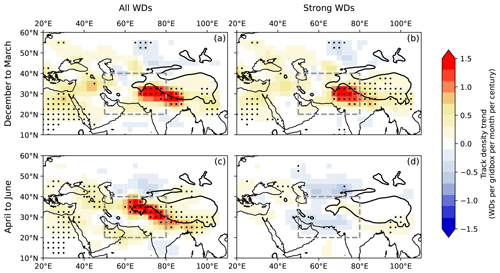
Figure 3Trends in WD frequency from 1950 to 2022. The number of unique tracks passing through each 2.5°×2.5° grid box per month is counted and aggregated by season, and the trend is computed. Two seasons are shown: (a, b) December to March and (c, d) April to June. To determine whether trends are sensitive to WD detection thresholds, trends for “strong WDs”, i.e. those whose peak 350 hPa ζ is in the top half of the population, are given in (b, d). Stippling indicates where trends have p<0.05. The dashed grey box (20–40° N, 50–80° E) indicates the aggregation region for WDs used throughout this study.
During the pre-monsoon months (Fig. 3c), this significant increasing trend persists across the western Himalaya and Hindu Kush but also extends over the Karakoram. Over north India, where WD frequencies are typically much lower during the pre-monsoon months than the winter, trends of similar magnitude to those seen in the winter (Fig. 3a) indicate a much bigger relative increase in WD frequency. This is potentially important for two reasons: firstly, as we have seen, these months are not typically well studied in the context of WD meteorology, and so trends here have thus far remained undiscovered, and secondly, this increases the chance of WDs coming into contact with the summer monsoon, which can lead to catastrophic flooding (Ranalkar et al., 2016; Hunt et al., 2021; Kalshetti et al., 2022).
There is no signal in the trend of stronger WDs during the pre-monsoon months (Fig. 3d), but this may be because intense WDs are uncommon during this time of year and so remain dominated by interannual variability. In fact, the intensity of WDs at this time of year may anyway matter less than during the winter, as such systems (particularly in or after June) have an increasingly ready supply of moisture from the advancing monsoon. For example, the July 2023 floods over north India which impacted a number of north Indian states, killing over a hundred people and resulting in extensive flooding in Delhi (Associated Press, 2023), were linked to the passage of a comparatively weak WD.
There is a weak but significant decline in the number of systems passing over central Asia, i.e. that are deflected north of the Tibetan Plateau, during the winter. These are already rare and have little impact on the weather of the western Himalaya, so we leave a more detailed investigation for later research.
In general, WD frequency trends follow the mean track density (Fig. 2), implying that there has probably not been a significant shift in regions impacted by WDs. It is also unlikely that studies reporting negative trends in WD frequency can be explained purely through their choice of region. As we will see, there have been nonzero trends in jet latitude over the study period, but as WDs often spin up locally (i.e. requiring the baroclinic environment provided by the orography), this seems to result in a change in frequency more than a change in location.
We now explore potential causes of these trends. It is known that both interannual and intraseasonal variability of WD frequency is strongly controlled by the latitude of the subtropical jet (Singh, 1971; Hunt et al., 2018a), so we can be fairly confident in a hypothesis that explains trends in WD frequency in terms of trends in upper-level zonal winds. Indeed, there has been a significant increase in the wind speed of the upper-level westerlies over northern India and the Himalaya over the last 70 years (Fig. 4).
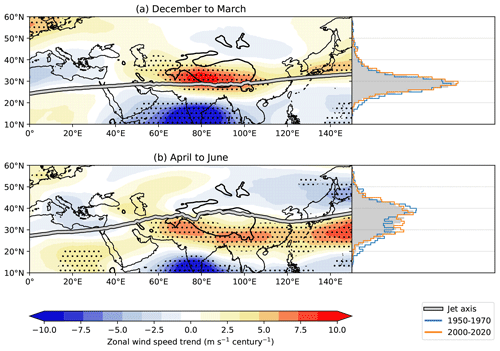
Figure 4Trends in 200 hPa seasonal mean zonal wind from 1950 to 2022. (a) December to March and (b) April to June. Stippling indicates where trends have p<0.05. The histograms on the right of each subplot show the latitudinal distribution of the jet axis for the respective season, computed as the latitude at which the monthly mean 200 hPa zonal wind reaches its peak when averaged across 50–80° E.
Changes in the subtropical jet are often linked to hemispheric-scale changes in the upper-level meridional temperature gradient (e.g. Stendel et al., 2021), but it is clear from the maximum over the Tibetan Plateau that there is also a locally forced change here as well. The sign of the trend and its local maximum here is consistent with earlier studies that have investigated the relationship between the winter subtropical jet and climate change over a similar period (e.g. Pena-Ortiz et al., 2013). This local response is crucial because it amplifies the effect of climate change on WDs, not only through modifying the jet but also by making the local environment more unstable (Krishnan et al., 2019). This locally amplified increase in subtropical jet strength could be caused by shifts in the Walker circulation (Liu et al., 2021) or trends in aerosol loading over north India (Abish et al., 2015; Chemke and Dagan, 2018), whereas the global-scale increase in the meridional gradient of tropical upper-tropospheric temperature is likely due to increased convection in the tropics (Menzel et al., 2019; Woollings et al., 2023).
The increasing trend in 200 hPa u is present in both the winter and pre-monsoon seasons and could thus explain increasing WD frequency in both seasons. However, larger mean u over north India could mean either that the jet is getting stronger or simply that it is more likely to be in this position. The histograms on the right of Fig. 4 showing the distribution of daily jet latitude for each season resolve this. In the winter months, the jet latitude distribution has a strong peak at 28° N with little variance. This largely coincides with the region of increasing u, although the trend maximum is located slightly to the north of the jet axis, implying that during the winter the jet has strengthened over recent decades. The distribution of jet latitudes has also shifted slightly northwards as we see from comparing the 1950–1970 and 2000–2020 distributions. In the pre-monsoon months, jet latitude varies considerably and is usually found between 30 and 40° N. This is largely to the north (though with some overlap) of the region of significantly increasing u, implying that in the pre-monsoon months the jet is more likely to be located further south in recent decades than previously. We can confirm this by looking at the change in jet latitude distribution from 1950–1970 to 2000–2020 (right side of Fig. 4b). In the latter period, the spring jet is much more likely to be found south of the Tibetan Plateau, and thus provide WDs to south Asia, than in the former period. Next, we will investigate the trends in jet strength and latitude more thoroughly, linking them directly to changes in WD behaviour.
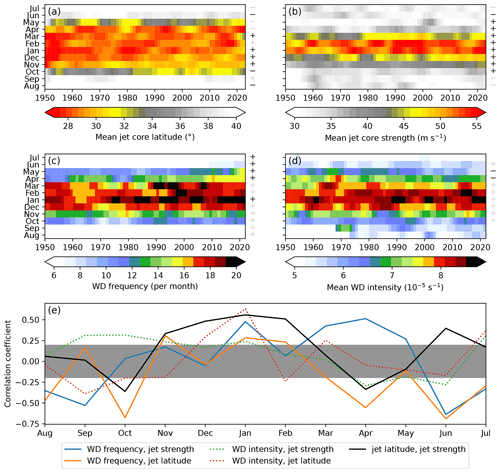
Figure 5Trends in selected WD and subtropical jet monthly statistics. Jet statistics are computed as an average between 20–40° N, 50–80° E. At the end of each row, a “+” indicates a positive trend and a “−” indicates a negative trend. These are coloured black and grey respectively for p<0.05 and p<0.5. (a) Latitude of the jet core, measured as the latitude where the zonal average of 200 hPa u is maximum. (b) Strength of the jet core, taken as the maximum of the zonal average of 200 hPa u (i.e. the zonal wind speed at the mean core location). (c) The number of unique WD tracks that enter the WD box within a given month. (d) The mean intensity of those WDs (again measured only within the box) taken as the 350 hPa ζ measured at the WD centre. (e) Pearson correlation coefficients between selected pairs of these statistics for each month. The grey band indicates where p>0.05. For (a)–(d), the annual time series have been smoothed with a 2-year Gaussian low-pass filter for clarity. However, only the original time series are used for computing statistics.
Part of the problem is that WDs respond to changes in the subtropical jet in different ways depending on the season. The most obvious example is the relationship between jet latitude and WD frequency. During the summer monsoon when the jet is far north, we would expect a negative correlation between jet latitude and WD frequency as anomalous southward excursions of the jet are more likely to bring WDs to north India. Conversely, in the winter months anomalous southward excursions of the jet move it away from the baroclinic environment of the Himalaya, reducing WD frequency and intensity (Baudouin et al., 2021).
For this reason, we now investigate the relationships between jet strength and latitude and WD frequency and intensity for each month (Fig. 5), allowing appropriate partitioning of effects into the winter and pre-monsoon months. The latitude of the jet core over south Asia has increased (i.e. moved poleward) in all months from November to March, with a significant trend in all months except February. Conversely, in the pre-monsoon and summer monsoon the jet has tended to a more southward position over the last 70 years, although only significantly so in June and August (Fig. 5a). This signal is particularly consequential during May and June as typically the jet starts to migrate northwards before or during the onset of the summer monsoon (Schiemann et al., 2009). The negative trends here imply that migration has become increasingly delayed in recent years. Jet core strength, on the other hand, has seen a significant increase in each month from October to May (Fig. 5b). This is in agreement with Fig. 4.
Within the WD box, monthly WD frequency has increased in every month over the last 70 years (Fig. 5c). However, the trends are only statistically significant in January and from April to July (i.e. through the whole pre-monsoon and monsoon onset). This, alongside substantial interdecadal variability, may explain why previous studies have disagreed on their assessments of WD frequency trends. We note, however (not shown), that moving to a 1979 start consistent with many studies (e.g. those based on older reanalyses) makes little difference to the sign or significance of the trends presented here. However, the increases in WD frequency after the winter seasons are particularly prominent and, in addition, appear to be recent phenomena. In May, prior to 2000 WDs occurred at the rate of about 10 per month, and this has risen to about 14 in the last 20 years; in June, they have risen from a rate of about 4 per month prior to 2000 to nearly 8 per month in the last 10 years.
For comparison, mean WD intensity has significantly declined in April and May, although the relative magnitude of this decline is small in the context of the population mean. Insignificant positive trends dominate the winter months. These results are consistent with Fig. 3, which showed that the frequency of WDs significantly increased in both winter and spring but that strong WDs increased only in winter (with no significant spring trend). Taken together, this implies that there is no significant trend in the intensity of winter WDs, and thus disagreements between studies confined to those months (i.e. December to March) cannot be explained by different choices of WD intensity threshold.
To help us causally connect the behaviour of the subtropical jet to WDs, we can also compute the annual cycles of correlations between selected pairs of these statistics (Fig. 5e). Firstly, jet characteristics are typically not a good predictor of monthly mean WD intensity, with which both jet strength and latitude are typically only weakly correlated. The exception is in the height of the winter (December and January) when WD intensity is significantly positively correlated with jet latitude. As we have already discussed, southward deviations of the jet from its mean position (about 28–30° N during these months) take WDs away from both mountain- and circulation-driven regions of baroclinic instability. Such WDs would still be detected but would not intensify as much as their counterfactuals.
The significant increase in January WD frequency can be attributed to increased subtropical jet strength over the last 70 years, consistent with Fig. 4a. A stronger jet offers deeper vertical wind shear and hence a greater source of baroclinic instability for WDs to form and grow (Sankar and Babu, 2021; Nischal et al., 2023). In January, therefore, WD frequency is predominantly related to jet strength, whereas WD intensity is predominantly related to jet position, although both jet statistics are significantly correlated with each of the WD statistics. This arises from both large-scale and local processes. On the synoptic scale, a stronger jet meanders more, resulting in a higher number of WDs at a specific location. In contrast, on the local scale, the precise positioning of the jet is what enables the meanders to grow into powerful WDs through interaction with the orography.
WDs have also increased significantly in every month from April to July, covering much of the pre-monsoon and monsoon seasons. In April this appears to have been driven by the sharp increase in jet strength, whereas in June and July it appears to be driven by a more equatorward positioning of the jet. We can probably rule out jet strength as a confounding factor in these months because the only known mechanism through which a weaker jet produces more WDs is through a latitudinal shift in its position, otherwise decreased baroclinicity would cause a decline in frequency. Nor is it the case that temporal averaging of a wavier jet makes it appear more weak – there is no significant positive trend in metrics of jet waviness in these transition months (not shown). May appears to represent a transitional period between the effects of jet latitude and jet strength.
In summary, WD frequency has significantly increased over the last 70 years in January and in April through July. In January and April we attribute this to increasing jet strength; in June and July we attribute this to decreasing jet latitude – in other words, the jet is more likely to remain over northern India and Pakistan as the monsoon develops, rather than receding northwards as it typically did in the second half of the twentieth century.
Western disturbances (WDs) are synoptic-scale cyclonic circulations that propagate westward along the subtropical jet, bringing heavy precipitation to Pakistan and northern India, particularly to the mountainous regions therein. They are a vital component of the region's water security, recharging glaciers and the snowpack as well as providing rainfall for irrigation at lower elevations. Although they are most common in winter (December to March), they can occur, albeit unusually, at any time of year. In rare cases, strong WDs can interact with the summer monsoon, producing catastrophically heavy rainfall.
Given this, it is important to quantify trends in WD frequency and intensity so that adequate preparations can be made. However, previous studies have largely disagreed on the sign and significance of WD frequency trends. In this study we took a more robust approach, breaking down the trends both spatially and seasonally using 70 years of reanalysis data.
Key results are as follows:
-
As previously found in Hunt et al. (2018a), WDs, as measured by their 350 hPa relative vorticity, are much stronger in winter months. WDs whose peak intensity exceeds the 75th percentile virtually never occur between June and September.
-
WD track density varies considerably between the winter and pre-monsoon months (here taken as April to June). During the winter, tracks predominantly impact the Hindu Kush and western Himalaya and can often persist long enough to reach the central and eastern Himalaya. During the pre-monsoon months, track density is more spread with many more impacting the Karakoram and relatively few impacting the central or eastern Himalaya.
-
WDs have increased significantly over the western Himalaya and Hindu Kush in winter and significantly over these regions and the Karakoram in the pre-monsoon months and early monsoon. As these approximately follow mean track density, there has been no marked shift in seasonal track density, nor do any regions show a significant decline in WD frequency.
-
Upper-level zonal wind has increased significantly over north India and Pakistan in both the winter and pre-monsoon seasons. This implies the subtropical jet has increased in intensity in the winter and is more frequently situated over the region during the pre-monsoon and early monsoon. Trend maxima over the Tibetan Plateau imply a strong local contribution to the forcing in circulation.
-
Over a box incorporating most of their population (20–40° N, 50–80° E), WDs have significantly increased in frequency in January, April, May, June, and July. Increases in May and June over the last 20 years have been particularly notable – for example, WDs in June are now twice as frequent as they were for much of the twentieth century. This implies a considerable lengthening of the WD season and therefore a significantly increased risk of WDs interacting with the summer monsoon.
-
We attributed the January and April frequency increases to a strengthening of the subtropical jet and the June and July increases to a delayed northward retreat of the jet.
-
Trends in WD intensity are statistically significant only in April and May, where they have declined slightly. We were not able to link these to changes in jet behaviour, and so this remains an open question.
This changing seasonality of WD frequency does not wholly explain the disagreement between earlier studies in establishing the sign and significance of historical WD frequency trends. Regional variations in these trends do not appear to explain why some studies have reported negative trends in WD frequency but do partially explain the mix of no, insignificantly positive, and significantly positive trends reported in previous studies, as the region of significantly positive trend in winter WD frequency reported here covers only the Hindu Kush and western and central Himalaya but not the Karakoram, a region often included in such studies. There has been no significant spatial shift in WD track density in the last 70 years, and so it is unlikely that this is the cause of previous disagreement.
One possible resolution which has not been explored here is the role of interdecadal variability. For example, WD frequency is known to be correlated with the North Atlantic Oscillation (Yadav et al., 2009; Filippi et al., 2014; Hunt and Zaz, 2023), and correlating monthly mean sea surface temperatures (SSTs) with WD frequency also suggests strong modulation by the Pacific Decadal Oscillation (not shown). Even if there is a long-term trend, which the analysis presented here supports, shorter study periods sampling different parts of the interdecadal variability would lead to conflicting answers.
Aside from this, an important shortcoming of this paper is the dependence on a single reanalysis. Not only should trends drawn from reanalyses be interpreted cautiously due to changes in observing systems (Thorne and Vose, 2010), but the reader should also be aware that tracked datasets drawn from different reanalyses can differ considerably (e.g. for extratropical cyclones; Hodges et al., 2011). It is also worth noting that the results presented here are somewhat at odds with those previously presented by the author (Hunt et al., 2019b), in which a similar method applied to Coupled Model Intercomparison Project Phase 5 (CMIP5) models indicated a significant decline in WD frequency and intensity, attributed to weakening baroclinicity. A more thorough review using CMIP6 model output is required.
ERA5 data were downloaded from https://doi.org/10.24381/cds.bd0915c6 (Hersbach et al., 2023) where they are freely available. The western disturbance track data are freely available on Zenodo at https://doi.org/10.5281/zenodo.8208018 (Hunt, 2023).
The author has declared that there are no competing interests.
Publisher's note: Copernicus Publications remains neutral with regard to jurisdictional claims made in the text, published maps, institutional affiliations, or any other geographical representation in this paper. While Copernicus Publications makes every effort to include appropriate place names, the final responsibility lies with the authors.
Kieran M. R. Hunt is supported by a NERC Independent Research Fellowship (MITRE; NE/W007924/1). Kieran M. R. Hunt also wishes to thank Jean-Philippe Baudouin, a discussion with whom inspired this paper.
This research has been supported by the National Centre for Earth Observation (grant no. NE/W007924/1).
This paper was edited by Nili Harnik and reviewed by Jean-Philippe Baudouin and A. P. Dimri.
Abish, B., Joseph, P. V., and Johannessen, O. M.: Climate change in the subtropical jetstream during 1950–2009, Adv. Atmos. Sci., 32, 140–148, 2015. a, b
Ahmad, I. and Sadiq, N.: Spatio-Temporal analysis of Western Disturbances over Pakistan, Nucleus, 49, 329–338, 2012. a
Ahmed, M., Das, B., Lotus, S., and Ali, M.: A study on frequency of western disturbances and precipitation trends over Jammu & Kashmir, India: 1980–2019, Mausam, 73, 283–294, 2022. a
Archer, D. R. and Fowler, H. J.: Spatial and temporal variations in precipitation in the Upper Indus Basin, global teleconnections and hydrological implications, Hydrol. Earth Syst. Sci., 8, 47–61, https://doi.org/10.5194/hess-8-47-2004, 2004. a
Associated Press: Record monsoon rains have killed more than 100 people in northern India over two weeks – apnews.com, https://apnews.com/article/india-monsoon-floods-deaths-8185e8e5b05fe9de76efc609928ec360 (last access: 31 July 2023), 2023. a, b
Baudouin, J.-P., Herzog, M., and Petrie, C. A.: Synoptic processes of winter precipitation in the Upper Indus Basin, Weather Clim. Dynam., 2, 1187–1207, https://doi.org/10.5194/wcd-2-1187-2021, 2021. a
Benn, D. I. and Owen, L. A.: The role of the Indian summer monsoon and the mid-latitude westerlies in Himalayan glaciation: review and speculative discussion, J. Geol. Soc., 155, 353–363, 1998. a
Cannon, F., Carvalho, L. M. V., Jones, C., and Bookhagen, B.: Multi-annual variations in winter westerly disturbance activity affecting the Himalaya, Clim. Dynam., 44, 441–455, 2015. a
Cannon, F., Carvalho, L. M. V., Jones, C., and Norris, J.: Winter westerly disturbance dynamics and precipitation in the western Himalaya and Karakoram: a wave-tracking approach, Theor. Appl. Climatol., 125, 27–44, 2016. a
Chemke, R. and Dagan, G.: The effects of the spatial distribution of direct anthropogenic aerosols radiative forcing on atmospheric circulation, J. Climate, 31, 7129–7145, 2018. a
Chevuturi, A. and Dimri, A. P.: Investigation of Uttarakhand (India) disaster-2013 using weather research and forecasting model, Nat. Hazards, 82, 1703–1726, 2016. a
Das, M. R., Mukhopadhyay, R. K., Dandekar, M. M., and Kshirsagar, S. R.: Pre-monsoon western disturbances in relation to monsoon rainfall, its advancement over NW India and their trends, Curr. Sci., 82, 1320–1321, 2002. a
De, U. S., Dube, R. K., and Rao, G. S. P.: Extreme weather events over India in the last 100 years, J. Ind. Geophys. Union, 9, 173–187, 2005. a
de Kok, R. J., Tuinenburg, O. A., Bonekamp, P. N. J., and Immerzeel, W. W.: Irrigation as a potential driver for anomalous glacier behavior in High Mountain Asia, Geophys. Res. Lett., 45, 2047–2054, 2018. a
Dimri, A. P. and Chevuturi, A.: Model sensitivity analysis study for western disturbances over the Himalayas, Meteorol. Atmos. Phys., 123, 155–180, 2014. a
Dimri, A. P. and Niyogi, D.: Regional climate model application at subgrid scale on Indian winter monsoon over the western Himalayas, Int. J. Climatol., 33, 2185–2205, 2012. a
Dimri, A. P., Niyogi, D., Barros, A. P., Ridley, J., Mohanty, U. C., Yasunari, T., and Sikka, D. R.: Western disturbances: a review, Rev. Geophys., 53, 225–246, 2015. a, b
Filippi, L., Palazzi, E., von Hardenberg, J., and Provenzale, A.: Multidecadal variations in the relationship between the NAO and winter precipitation in the Hindu Kush–Karakoram, J. Climate, 27, 7890–7902, 2014. a
Ganju, A. and Dimri, A. P.: Prevention and mitigation of avalanche disasters in western Himalayan region, Nat. Hazards, 31, 357–371, 2004. a
Hersbach, H., Bell, B., Berrisford, P., Hirahara, S., Horányi, A., Muñoz-Sabater, J., Nicolas, J., Peubey, C., Radu, R., Schepers, D., Simmons, A., Soci, C., Abdalla, S., Abellan, X., Balsamo, G., Bechtold, P., Biavati, G., Bidlot, J., Bonavita, M., De Chiara, G., Dahlgren, P., Dee, D., Diamantakis, M., Dragani, R., Flemming, J., Forbes, R., Fuentes, M., Geer, A., Haimberger, L., Healy, S., Hogan, R. J., Hólm, E., Janisková, M., Keeley, S., Laloyaux, P., Lopez, P., Lupu, C., Radnoti, G., de Rosnay, P., Rozum, I., Vamborg, F., Villaume, S., and Thépaut, J.-N.: The ERA5 global reanalysis, Q. J. Roy. Meteorol. Soc., 146, 1999–2049, 2020. a
Hersbach, H., Bell, B., Berrisford, P., Biavati, G., Horányi, A., Muñoz Sabater, J., Nicolas, J., Peubey, C., Radu, R., Rozum, I., Schepers, D., Simmons, A., Soci, C., Dee, D., and Thépaut, J.-N.: ERA5 hourly data on pressure levels from 1940 to present, Copernicus Climate Change Service (C3S) Climate Data Store (CDS) [data set], https://doi.org/10.24381/cds.bd0915c6, 2023. a
Hewitt, K.: The Karakoram anomaly? Glacier expansion and the `elevation effect', Karakoram Himalaya, Mount. Res. Dev., 25, 332–340, 2005. a
Hodges, K. I., Lee, R. W., and Bengtsson, L.: A comparison of extratropical cyclones in recent reanalyses ERA-Interim, NASA MERRA, NCEP CFSR, and JRA-25, J. Climate, 24, 4888–4906, 2011. a
Hunt, K. M. and Zaz, S. N.: Linking the North Atlantic Oscillation to winter precipitation over the Western Himalaya through disturbances of the subtropical jet, Clim. Dynam., 60, 2389–2403, 2023. a, b
Hunt, K. M. R.: Tracks of western disturbances (1950–2022) impacting South Zenodo [data set], https://doi.org/10.5281/zenodo.8208018, 2023. a
Hunt, K. M. R. and Dimri, A. P.: Synoptic-scale precursors of landslides in the western Himalaya and Karakoram, Sci. Total Environ., 776, 145895, https://doi.org/10.1016/j.scitotenv.2021.145895, 2021. a
Hunt, K. M. R., Turner, A. G., and Shaffrey, L. C.: The evolution, seasonality, and impacts of western disturbances, Q. J. Roy. Meteorol. Soc., 144, 278–290, https://doi.org/10.1002/qj.3200, 2018a. a, b, c, d, e
Hunt, K. M. R., Turner, A. G., and Shaffrey, L. C.: Extreme daily rainfall in Pakistan and north India: scale-interactions, mechanisms, and precursors, Mon. Weather Rev., 146, 1005–1022, 2018b. a
Hunt, K. M. R., Turner, A. G., and Shaffrey, L. C.: Representation of western disturbances in CMIP5 models, J. Climate, 32, 1997–2011, https://doi.org/10.1175/JCLI-D-18-0420.1, 2019a. a
Hunt, K. M. R., Turner, A. G., and Shaffrey, L. C.: Falling trend of western disturbances in future climate simulations, J. Climate, 32, 5037–5051, 2019b. a
Hunt, K. M. R., Turner, A. G., and Schiemann, R. K. H.: How interactions between tropical depressions and western disturbances affect heavy precipitation in South Asia, Mon. Weather Re., 149, 1801–1825, 2021. a, b
Javed, A., Kumar, P., Hodges, K. I., Sein, D. V., Dubey, A. K., and Tiwari, G.: Does the recent revival of Western Disturbances govern the Karakoram Anomaly?, J. Climate, 35, 4383–4402, 2022. a
Kalshetti, M., Chattopadhyay, R., Hunt, K. M. R., Phani, R., Joseph, S., Pattanaik, D. R., and Sahai, A. K.: Eddy transport, wave–mean flow interaction, and Eddy forcing during the 2013 Uttarakhand extreme event in the reanalysis and S2S retrospective forecast data, Int. J. Climatol., 42, 8248–8268, 2022. a
Kotal, S. D., Roy, S. S., and Roy Bhowmik, S. K.: Catastrophic heavy rainfall episode over Uttarakhand during 16–18 June 2013 – observational aspects, Curr. Sci., 107, 234–245, 2014. a
Krishnan, R., Sabin, T. P., Madhura, R. K., Vellore, R. K., Mujumdar, M., Sanjay, J., Nayak, S., and Rajeevan, M.: Non-monsoonal precipitation response over the Western Himalayas to climate change, Clim. Dynam., 52, 4091–4109, 2019. a, b
Kumar, N., Yadav, B. P., Gahlot, S., and Singh, M.: Winter frequency of western disturbances and precipitation indices over Himachal Pradesh, India: 1977–2007, Atmósfera, 28, 63–70, 2015. a
Lanzante, J. R., Klein, S. A., and Seidel, D. J.: Temporal homogenization of monthly radiosonde temperature data. Part I: Methodology, J. Climate, 16, 224–240, 2003. a
Liu, X., Grise, K. M., Schmidt, D. F., and Davis, R. E.: Regional characteristics of variability in the Northern Hemisphere wintertime polar front jet and subtropical jet in observations and CMIP6 models, J. Geophys. Res.-Atmos., 126, e2021JD034876, https://doi.org/10.1029/2021JD034876, 2021. a
Madhura, R. K., Krishnan, R., Revadekar, J. V., Mujumdar, M., and Goswami, B. N.: Changes in western disturbances over the Western Himalayas in a warming environment, Clim. Dynam., 44, 1157–1168, 2015. a
Menzel, M. E., Waugh, D., and Grise, K.: Disconnect between Hadley cell and subtropical jet variability and response to increased CO2, Geophys. Res. Lett., 46, 7045–7053, 2019. a
Midhuna, T. M., Kumar, P., and Dimri, A. P.: A new Western Disturbance Index for the Indian winter monsoon, J. Earth Syst. Sci., 129, 59, https://doi.org/10.1007/s12040-019-1324-1, 2020. a
Mooley, D. A.: The role of western disturbances in the production of weather over India during different seasons, Ind. J. Meteorol. Geophys., 8, 253–260, 1957. a
Neal, R., Robbins, J., Dankers, R., Mitra, A., Jayakumar, A., Rajagopal, E. N., and Adamson, G.: Deriving optimal weather pattern definitions for the representation of precipitation variability over India, Int. J. Climatol., 40, 342–360, 2020. a
Nischal, S., Attada, R., and Hunt, K. M. R.: Evaluating winter precipitation over the western Himalayas in a high-resolution Indian regional reanalysis using multisource climate datasets, J. Appl. Meteorol. Clim., 61, 1613–1633, 2022. a
Nischal, S., Rohtash, K. S., Pathaikara, A., Punde, P., and Attada, R.: Hydrological Extremes in Western Himalayas-Trends and Their Physical Factors, in: Natural Hazards – New Insights, Chap. 20, edited by: Mokhtari, D. M., IntechOpen, Rijeka, https://doi.org/10.5772/intechopen.109445, 2023. a
Nischal, S., Attada, R., and Hunt, K. M. R.: Evaluating winter precipitation over the Western Himalayas in high-resolution Indian regional reanalysis using multi-source climate datasets, J. Appl. Meteorol. Clim., 61, 1613–1633, 2021. a
Pena-Ortiz, C., Gallego, D., Ribera, P., Ordonez, P., and Alvarez-Castro, M. D. C.: Observed trends in the global jet stream characteristics during the second half of the 20th century, J. Geophys. Res.-Atmos., 118, 2702–2713, 2013. a
Pisharoty, P. R. and Desai, B. N.: Western disturbances and Indian weather, Ind. J. Meteorol. Geophys., 7, 333–338, 1956. a
Ranalkar, M. R., Chaudhari, H. S., Hazra, A., Sawaisarje, G., and Pokhrel, S.: Dynamical features of incessant heavy rainfall event of June 2013 over Uttarakhand, India, Nat. Hazards, 80, 1579–1601, 2016. a
Rao, Y. P. and Srinivasan, V.: Discussion of typical synoptic weather situation: winter western disturbances and their associated features, Tech. Rep., IMD Forecasting Manual Report-III 1.1, India Meteorological Department, https://www.imdpune.gov.in/Reports/Forecasting_Mannuals/IMD_III-3.8.pdf (last access: 28 February 2024), 1969. a, b
Sankar, N. V. and Babu, C. A.: Study on Environmental and Thermodynamic Factors that influence Precipitation associated with Western Disturbances over North India during Winter, Pure Appl. Geophys., 178, 4073–4096, 2021. a
Schiemann, R., Lüthi, D., and Schär, C.: Seasonality and interannual variability of the westerly jet in the Tibetan Plateau region, J. Climate, 22, 2940–2957, 2009. a, b
Shekhar, M. S., Chand, H., Kumar, S., Srinivasan, K., and Ganju, A.: Climate-change studies in the western Himalaya, Ann. Glaciol., 51, 105–112, 2010. a
Singh, M. S.: The study of the jet stream over India and to its north in winter, Part-I, Ind. J. Meteorol. Geophys., 22, 1–14, 1971. a, b
Singh, M. S. and Agnihotri, C. L.: Baroclinity over India in winter and its relation to western disturbances and jet streams. I, Ind. J. Meteorol. Hydrol. Geophys., 28, 303–310, 1977. a
Smith, D., Dorling, S., Renfrew, I., Ross, A., and Poku, C.: Fog trends in India: relationships to fog type and western disturbances, Int. J. Climatol., 43, 818–836, 2023. a
Stendel, M., Francis, J., White, R., Williams, P. D., and Woollings, T.: The jet stream and climate change, in: Climate change, Elsevier, 327–357, https://doi.org/10.1016/B978-0-12-821575-3.00015-3, 2021. a
Thayyen, R. J. and Gergan, J. T.: Role of glaciers in watershed hydrology: a preliminary study of a “Himalayan catchment”, The Cryosphere, 4, 115–128, https://doi.org/10.5194/tc-4-115-2010, 2010. a
Thorne, P. W. and Vose, R. S.: Reanalyses suitable for characterizing long-term trends, B. Am. Meteorol. Soc., 91, 353–362, 2010. a
Unnikrishnan, C. K., Pawar, S., and Gopalakrishnan, V.: Satellite-observed lightning hotspots in India and lightning variability over tropical South India, Adv. Space Res., 68, 1690–1705, 2021. a
Valdiya, K. S.: Anomalous weather events in central Himalaya: real-world observations and some questions, Curr. Sci., 119, 19–25, https://doi.org/10.18520/cs/v119/i1/19-25, 2020. a
Woollings, T., Drouard, M., O'Reilly, C. H., Sexton, D. M., and McSweeney, C.: Trends in the atmospheric jet streams are emerging in observations and could be linked to tropical warming, Commun. Earth Environ., 4, 125, https://doi.org/10.1038/s43247-023-00792-8, 2023. a
Yadav, R. K., Rupa Kumar, K., and Rajeevan, M.: Increasing influence of ENSO and decreasing influence of AO/NAO in the recent decades over northwest India winter precipitation, J. Geophys. Res.-Atmos., 114, D12112, https://doi.org/10.1029/2008JD011318, 2009. a
Yadav, R. K., Kumar, K. R., and Rajeevan, M.: Characteristic features of winter precipitation and its variability over northwest India, J. Earth Syst. Sci., 121, 611–623, 2012. a
Yuval, J. and Kaspi, Y.: Eddy activity response to global warming–like temperature changes, J. Climate, 33, 1381–1404, 2020. a